COMING SOON SPRING 2025
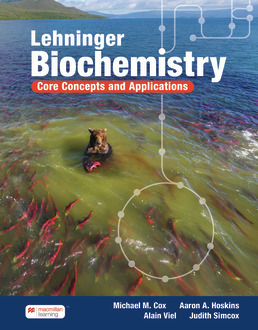
Lehninger Biochemistry: Core Concepts and Applications
First Edition ©2025 Michael M. Cox; Aaron A. Hoskins; Alain Viel; Judith Simcox Formats: Achieve, E-book, Print
As low as $124.99
As low as $124.99
Authors
-
Michael Cox
Michael M. Cox was born in Wilmington, Delaware. In his first biochemistry course, the first edition of Lehninger's Biochemistry was a major influence in refocusing his fascination with biology and inspiring him to pursue a career in biochemistry. After graduate work at Brandeis University with William P. Jencks and post-doctoral work at Stanford with I. Robert Lehman, he moved to the University of Wisconsin–Madison in 1983. He became a full professor of Biochemistry in 1992.
Mike Cox has coordinated an active research team at Wisconsin investigating the function and mechanism of enzymes that act at the interface of DNA replication, repair, and recombination. That work has resulted in over 200 publications to date.
For more than three decades, Cox has taught introductory biochemistry to undergraduates and has lectured in a variety of graduate courses. He organized a course on professional responsibility for first-year graduate students and established a systematic program to draw talented biochemistry undergraduates into the laboratory at an early stage of their college career. He has received multiple awards for both his teaching and his research, including the Eli Lilly Award in Biological Chemistry, election as an AAAS fellow, and the UW Regents Teaching Excellence Award. Cox's hobbies include turning 18 acres of Wisconsin farmland into an arboretum, wine collecting, and assisting in the design of laboratory buildings.
-
Aaron Hoskins
Aaron A. Hoskins was born in Lafayette, Indiana, received his BS in chemistry from Purdue in 2000, and earned his PhD in biological chemistry at Massachusetts Institute of Technology with JoAnne Stubbe. In 2006, he went to Brandeis and University of Massachusetts Medical School as a postdoctoral fellow with Melissa Moore and Jeff Gelles. Hoskins joined the University of Wisconsin–Madison biochemistry faculty in 2011.
Hoskins's PhD research was on de novo purine biosynthesis. At Brandeis and University of Massachusetts, he began to study eukaryotic pre-mRNA splicing. During this time, he developed new single-molecule microscopy tools for studying the spliceosome.
Hoskins's laboratory is focused on understanding how spliceosomes are assembled and regulated and how they recognize introns. Hoskins has won awards for his research, including being named a Beckman Young Investigator and Shaw Scientist. He has taught introductory biochemistry for undergraduates since 2012. Hoskins also enjoys playing with his cat (Louise) and dog (Agatha), yoga/exercise, and tries to read a new book each week.
-
Alain Viel
Alain Viel a native of Vesoul (France), earned his PhD in developmental Biology from the University of Paris VI (France), studying under the guidance of Prof. Hermann Denis and Marc le Maire. His research focused on the storage of proteins and RNAs during amphibian oogenesis and using biochemical approaches. As a postdoctoral fellow in Prof. Daniel Branton's lab at Harvard University, he made significant strides in understanding the structure-function relationship of spectrin and the role of spliced variants of the tumor suppressor Hdlg.
In 2004, Viel became a senior lecturer in the Department of Molecular and Cellular Biology at Harvard University. He created LS100, a course where Harvard undergraduate students experience how science is done through the practice of experimental inquiry. His interest in developing accessible teaching material prompted Viel to create a blended-learning biochemistry course (MCB63) concomitantly with a version of the course for HarvardX (MCB63X). He has also developed an online course introducing non-scientists to CRISPR and its application in the life sciences.
Viel is the faculty co-director of LabXchange, an educational platform created at Harvard University. LabXchange offers an integrated teaching and learning ecosystem, engages with a diverse, global community, and distributes free high-quality STEM educational material, allowing everyone, everywhere, to chart a path in science.
Viel's influence extends beyond the classroom. As a founding member of BioVisions, a collaboration between scientists, teaching faculty, students, and media professionals, he has contributed to science visualization on a global scale. His collaborations with Prof. Lue have resulted in award-winning animations depicting biological processes in the context of the cell. These animations are widely used in high schools, colleges, museums, and TV series across the United States and Europe.
Viel was awarded the UNESCO Chair on Life Sciences and Social Innovation in 2023 for his contributions to digital education.
-
Judith Simcox
Judith Simcox grew up in Montana, earning her PhD in Biochemistry from the University of Utah studying with Dr. Donald McClain. As a graduate student, her research focused on circadian regulation of glucose metabolism. She then moved to studying the regulation of energy expenditure by lipid processing in her postdoctoral fellowship in the lab of Dr. Claudio Villanueva.
In 2019, Simcox became an Associate Professor of Biochemistry at the University of Wisconsin-Madison exploring inter-organ communication through lipid derived metabolites. By focusing on the lipids in the blood plasma, the Simcox lab asks the basic questions of: what tissues are producing these lipids and what is their function? The goal of this work is to understand how these lipids are functioning to drive metabolic diseases including cardiovascular disease, type 2 diabetes, and Alzheimer's disease. This work has won Simcox several awards including the 2023 American Society for Biochemistry and Molecular Biology (ASBMB) Walter A Shaw Young Investigator Award in Lipid Research, 2022 Journal of Lipid Research (JLR) Rising Star in Lipid Research Award, and selection as a Howard Hughes Medical Institute Freeman Hrabowski Scholar.
Simcox teaches biochemistry for undergraduates since 2019, focusing on modules to explore metabolism. She is a nationally recognized leader in building programs to increase access to science for her work with the Native American Research Internship at the University of Utah, and the Center for Indigenous Research to Create Learning and Excellence at the University of Wisconsin-Madison. In her free time, Simcox enjoys walks with her dogs (Roo, Ginger, Barney), bird watching, and running.
Table of Contents
Chapter 1: Biochemistry Concepts and Themes
1.1 Science and the Scientific Method
- What is Science?
- What is the Scientific Method?
1.2 Organisms, Cells, Chromosomes, and Genes
- Organisms Belong to Three Distinct Domains of Life
- Cells Are the Structural and Functional Units of All Living Organisms
- Viruses Cannot Live Independently of Cells
- Bacterial Cells Feature a Relatively Simple Architecture and Streamlined Lifestyles
- Eukaryotic Cells Have a Variety of Membranous Organelles
- Cells Contain a Wide Range of Supramolecular Structures
- Major Model Organisms and Systems are Useful in Biochemistry
- The Linear Sequence in DNA Encodes Proteins with Three-Dimensional Structures
1.3 The Organic Chemistry of Biochemistry
- Major Organic Species are Found in Cells
- Macromolecules Are the Major Constituents of Cells
- Molecular Weight and Molecular Mass are Expressed by Distinct Conventions
- Nucleophiles and Electrophiles Define How Many Reactions Proceed
- Cofactors Facilitate Particular Classes of Biochemical Reactions
1.4 A Review of Basic Thermodynamics
- Equilibrium Constants and Rate Constants Describe Distinct but Related Thermodynamic Parameters
- Organisms Transform Energy and Matter from Their Surroundings
- Creating and Maintaining Order Requires Work and Energy
1.5 Using Data Banks
Chapter 2: Water: The Chemistry of Life
2.1 Weak Interactions in Aqueous Systems
- Hydrogen Bonds Give Water Its Unusual Properties
- Water Interacts Electrostatically with Charged Solutes
- Nonpolar Gases Are Poorly Soluble in Water
- The Hydrophobic Effect is an Entropy-based Phenomenon
- van der Waals Interactions and Other Weak Interactions Are Key to Macromolecular Structure and Function
2.2 Ionization of Water, Weak Acids, and Weak Bases
- The Ionization of Water Is Expressed by an Equilibrium Constant
- The pH Scale Designates H+ and OH– Concentrations
- Weak Acids and Bases Have Characteristic Acid Dissociation Constants
- Titration Curves Reveal the pKa of Weak Acids
2.3 Buffering against pH Changes in Biological Systems
- A Buffer System Resists Changes in pH in Response to Added Acid or Base.
- The Henderson-Hasselbalch Equation Relates pH, pKa, and Buffer Concentration
- Weak Acids or Bases Buffer Cells and Tissues against pH Changes
- Phosphate and Bicarbonate Are Important Biological Buffer Systems Untreated Diabetes Produces Life-Threatening Acidosis
Chapter 3: Amino Acids, Peptides, and Proteins
3.1 Amino Acids
- What is an Amino Acid?
- The Amino Acid Residues in Proteins Are L Stereoisomers
- Amino Acids Can Be Classified by R Group
- Some Amino Acids Absorb Ultraviolet Light
- Uncommon Amino Acids Also Have Important Functions
- Amino Acids Can Act as Acids and Bases
- Amino Acids Differ in Their Acid-Base Properties
3.2 Peptides and Proteins
- Peptides Are Chains of Amino Acids
- Disulfide Bonds Occur in Some Proteins
- Ionization Behavior Can Distinguish Peptides
- Some Proteins Contain Chemical Groups Other Than Amino Acids
3.3 Purifying Proteins
- Proteins Can Be Separated and Purified
- Proteins Are Detected and Quantified Based on Their Functions
- Proteins Can Be Separated and Characterized by Electrophoresis
3.4 The Primary Structure of Proteins and Protein Chemistry
- There are Levels of Complexity to Protein Structure
- The Function of a Protein Depends on Its Amino Acid Sequence
- There are Multiple Ways to Reduce a Polypeptide Chain into Fragments.
- Mass Spectrometry Provides Information on Molecular Mass, Amino Acid Sequence, and Entire Proteomes
- Amino Acid Sequences Provide Important Biochemical and Evolutionary Information
Chapter 4: Protein Structure
4.1 Forces and Interactions that Stabilize Protein Structures
- Protein Structures Are Largely Stabilized by Weak Interactions
- Hydrogen Bonding, Ion Pairs, and van der Waals Interactions Also Contribute to Protein Folding
- The Conformation of the Peptide Bond Constrains Polypeptide Conformation
4.2 Secondary Protein Structure
- The α Helix Maximizes the Use of Polypeptide Hydrogen Bonds
- The β Strand is a Common Secondary Structure with an Extended Conformation
- Ramachandran Plots Describe the Distribution of Secondary Structure in a Protein
4.3 Tertiary and Quaternary Protein Structure
- Fibrous Proteins Have a Single Type of Secondary Structure
- The Fibrous Protein Collagen is the Most Abundant Protein in Mammals
- Silk is Made from a Fibrous Protein with b-sheet Secondary Structure
- Globular Proteins are Compact and Highly Varied in Three Dimensional Structure
- Protein Tertiary Structures can be Described in Terms of Motifs and Domains.
- Intrinsically Disordered Proteins Lack Stable Tertiary Structures.
- Quaternary Structure Describes the Organization of Multisubunit Proteins.
- Biomolecular Structures Can be Determined Using a Variety of Methods
- The Protein Data Bank is a Repository for Biomolecular Structures
4.4 Protein Denaturation and Folding
- Loss of Protein Structure Results in Loss of Function
- Amino Acid Sequence Determines Tertiary Structure
- Protein Folding Occurs by Defined Pathways and can be Assisted by Chaperones.
- Defects in Protein Folding Cause Human Disease
Chapter 5: Protein Function and Ligand Binding
5.1 Reversible Protein-Ligand Binding
- Ligands Bind to Proteins Reversibly at Binding Sites
- Protein-Ligand Interactions Can Be Described Quantitatively
5.2 Reversible Binding of a Protein to a Ligand: Oxygen-Binding by Myoglobin
- Oxygen Can Bind to a Heme Prosthetic Group
- Globins Are a Family of Oxygen-Binding Proteins
- The Binding of Oxygen to Myoglobin can be Described Quantitatively
- Protein Structure Affects How Ligands Bind
5.3 Reversible and Cooperative Binding of a Protein to a Ligand: Oxygen-Binding by Hemoglobin
- Hemoglobin Subunits Are Structurally Similar to Myoglobin
- Hemoglobin Undergoes a Structural Change on Binding Oxygen
- Hemoglobin Binds Oxygen Cooperatively
- Cooperative Ligand Binding Can Be Described Quantitatively
- Hemoglobin Also Transports H+ and CO2
5.4 Medical Conditions Related to Hemoglobin
- CO Binding to Hemoglobin Poses a Serious Health Risk
- Altered Hemoglobin Subunit Interactions in Sickle Cell Anemia Cause Pain and Suffering
Chapter 6: Protein Function and Enzymes
6.1 What are Enzymes?
- Most Enzymes Are Proteins
- Enzyme-catalyzed Reactions Occur Within Active Sites
- Enzymes Affect Reaction Rates, Not Equilibria
- Reaction Rates and Equilibria are Described by Constants
6.2 How Enzymes Work
- Noncovalent Interactions between Enzyme and Substrate Are Optimized in the Transition State
- Enzymes Use a Variety of Additional Chemical Mechanisms to Facilitate Catalysis
- Coenzymes Facilitate Particular Types of Reactions
6.3 Enzyme Kinetics
- The Steady State of an Enzyme-catalyzed Reaction Reflects the Concentration of ES
- The Relationship Between Substrate Concentration and Reaction Rate can be Described Quantitatively
- Scientists Compare Enzymes Using Vmax and Km.
- Enzymes are Subject to Reversible and Irreversible Inhibition
6.4 Chymotrypsin and Enzymatic Catalysis
- The Chymotrypsin Mechanism Involves Acylation and Deacylation of an Active Site Ser Residue
- An Understanding of Protease Mechanisms Led to Treatments for HIV
- An Understanding of Enzyme Mechanism Leads to Useful Antibiotics
6.5 Regulatory Enzymes
- Some Enzymes are Regulated by Allosteric Conformational Changes in Response to Modulator Binding
- Some Enzymes are Regulated by Reversible Covalent Modification
- Some Enzymes are Regulated by Proteolytic Cleavage of an Enzyme Precursor
Chapter 7: Carbohydrates
7.1 Monosaccharides and Disaccharides
- The Two Families of Monosaccharides Are Aldoses and Ketoses
- The Common Monosaccharides Have Cyclic Structures
- Sugars Containing and Forming Aldehydes are Reducing Sugars
- Disaccharides Consist of Two Monosaccharides Joined by a Glycosidic Bond
7.2 Polysaccharides
- Some Homopolysaccharides Are Storage Forms of Fuel While Others have Structural Roles
- Glycosaminoglycans Are Heteropolysaccharides of the Extracellular Matrix
7.3 Glycoconjugates: Peptidoglycans, Proteoglycans, Glycoproteins, and Glycolipids
- Peptidoglycan Reinforces the Bacterial Cell Wall
- Proteoglycans Are Glycosaminoglycan-Containing Macromolecules of the Cell Surface and Extracellular Matrix
- Glycoproteins Are Proteins with Covalently Attached Oligosaccharides
- Glycolipids and Lipopolysaccharides Are Membrane Components
7.4 Carbohydrates as Signaling Molecules
- Oligosaccharides Have Highly Diverse Structures
- Lectins Are Proteins That Bind Specifically to Complex Oligosaccharides and Mediate Many Biological Processes
Chapter 8: Lipids, Membranes, and Membrane Proteins
8.1 Membrane Lipids
- Fatty Acids are the Hydrocarbon Chain of Membrane Lipids
- Fatty Acid Composition of Lipids Impacts Health
- Structural Elements Determine Membrane Classes
- Membranes Lipids are Amphipathic Molecules that Form Lipid Bilayers
- Membrane Lipid Composition Impacts Membrane Fluidity
8.2 The Architecture of Membrane Proteins
- Membrane Proteins Differ in How They Associate with the Membrane Bilayer
- Integral Membrane Proteins Span Membranes and Can be Transporters
- Peripheral Membrane Proteins Interact with Membranes through Electrostatic Charge
- Lipid-anchored Proteins are Covalently Linked to Hydrophobic Anchors Embedded in the Membrane
8.3 Moving Molecules Through Membranes
- Membrane Transporters are Required to Move Large and Charged Molecules across Membranes
- Transport in and out of Cells May be Passive or Active
- Transporters and Ion Channels Share Structural Properties but Have Different Mechanisms
- The Glucose Transporter of Erythrocytes Mediates Passive Transport
- P-Type ATPases are Active Transporters that Change Conformation with Phosphoryl- Group Transfer from ATP
- Ion Channels Allow Rapid Movement of Ions Across Membranes
Chapter 9: Nucleotides and Nucleic Acids
9.1 Nucleotides
- Nucleotides Have Three Molecular Components
- The Common Nucleotides Have Many Uncommon Variants
- Phosphodiester Bonds Link Successive Nucleotides in Nucleic Acids
- The Properties of Nucleotide Bases Affect the Three-Dimensional Structure of Nucleic Acids
9.2 Nucleic Acid Structures
- DNA Is a Double Helix That Stores Genetic Information
- DNA Can Occur in Different Three-Dimensional Forms
- Certain DNA and RNA Sequences Adopt Unusual Structures
- Messenger RNAs Code for Polypeptide Chains
- Many RNAs Have More Complex Three-Dimensional Structures
9.3 Nucleic Acid Chemistry
- Double-Helical DNA and RNA Can Be Denatured
- Base Stacking Affects the UV Absorption Properties of DNA and RNA
- Nucleotides and Nucleic Acids Undergo Nonenzymatic Transformations
9.4 Nucleotide Roles in Cell Energetics and Signaling
- Nucleotides Carry Chemical Energy in Cells
- Some Nucleotides Are Regulatory Molecules or Signals
- Adenine Nucleotides Serve as Constituents of Many Enzymatic Cofactors; a Clue to the Origin of Life?
Chapter 10: Biological Information Part 1: DNA and RNA Metabolism
10.1 DNA Replication
- DNA Replication Follows a Set of Rules
- DNA Polymerases Synthesizes DNA
- DNA Replication Requires Many Enzymes and Protein Factors
- DNA Replication Occurs in Stages
10.2 DNA Repair and Organization
- All Cells Have Multiple DNA Repair Systems
- DNA Repair Can Also Occur in the Absence of Replication
- DNA Is Organized into Chromatin
10.3 Transcription and RNA Processing
- RNA Polymerases Synthesizes RNA
- RNA Replication Requires Many Enzymes and Protein Factors
- RNA Syntheses Occurs in Stages
- Medicines can Target or Be Made by RNA Polymerases
- Nearly All Eukaryotic RNAs Must Be Processed
- Reverse Transcriptases Produce DNA From RNA
10.4 Regulation of Transcription
- Transcription of Specific Genes Requires Regulatory Proteins in Addition to RNA Polymerase
- Regulation of Gene Expression in Bacteria
- Regulation of Gene Expression in Eukaryotes
Chapter 11: Biological Information Part 2: Protein Metabolism
11.1 The Genetic Code
- The Genetic Code Describes How Sets of Nucleic Acids Correspond to Particular Amino Acids
- tRNA Anticodons Base Pair with Codons
- tRNAs are Charged with Amino Acids for Protein Synthesis
- tRNA Charging Requires ATP Hydrolysis
11.2 Structure and Function of Ribosomes
- Ribosomes Catalyze Protein Synthesis
- Protein Synthesis Occurs in Stages
- Translation Factors Interact with the Ribosome During Elongation and Termination
- Protein Synthesis by the Ribosomes is Energetically Expensive
11.3 Protein Folding, Modification, and Degradation
- Chaperones Help Proteins Fold into Their Native Conformation
- Posttranslational Modifications are Critical for the Function of Many Proteins
- Protein Degradation is Highly Regulated in Eukaryotes by the Ubiquitin/Proteosome Pathway
11.4 Translational Control
- Riboswitches, Small RNAs, and Attenuation Can Control Gene Expression in Bacteria
- Eukaryotes Use mRNA Binding Proteins, RNAi, and MicroRNAs to Regulate Protein Production
Chapter 12: Nucleic Acid Technologies
12.1 Defining Genomic Information
- The Genome is All of the Nucleic Acid Needed to Support the Life of an Organism
- The Polymerase Chain Reaction Provides Targeted Amplification of Genomic Information
- DNA Can Be Sequenced
- Sanger Sequencing has been Automated
- Next-Generation DNA Sequencing Produces Complete Genome Sequences
- RNA Can be Sequenced by First Copying the RNA to DNA with Reverse Transcriptase
12.2 Altering Genomic Information
- Joining DNA Segments from Different Sources Yields Recombinant DNA Segments Can be Joined Without Using Restriction Enzymes
- Cloned DNA Can be Altered to Study Genes and Proteins
- CRISPR/Cas Systems Allows Targeted Cleavage or Modification of Genomic Information
12.3 Using Genomic Information
- An Altered Genome can Lead to an Altered Transcriptome and Proteome
- Genomic Information Can be Used to Identify the Source of Genetic Diseases
- Genomic Information Can be Used to Investigate Crimes
Chapter 13: Introduction to Intermediary Metabolism
13.1 What is Metabolism?
- Molecules are Metabolized by Anabolic and Catabolic Pathways
- Metabolic Pathways can be Converging, Diverging, or Cyclic
13.2 Common Enzyme Reactions in Metabolism
- Carbonyls are Important for Making and Breaking Carbon–Carbon Bonds
- Rearrangement and Isomerization Reactions Reposition Reactive Groups
- Elimination Reactions Release Good Leaving Groups
- Free-Radical Reactions Involve Complex Rearrangements
- Group Transfer Reactions Add or Subtract Functional Groups to Metabolites
- Oxidation-Reduction Reactions Involve Electron Transfer to or from Biomolecules
13.3 ATP and Phosphoryl Group Transfers
- ATP Contains High Energy Phosphodiester Bonds
- ATP Hydrolysis is Thermodynamically Very Favorable
- Many Other Metabolites and Enzyme Reaction Intermediates Also Have Large, Negative Free Energies of Hydrolysis
- ATP Donates Phosphoryl, Pyrophosphoryl, and Adenyl Groups
- ATP can Provide Energy by Group Transfers, Not Just by Hydrolysis
13.4 Biological Oxidation-Reduction Reactions
- Oxidation-Reduction Reactions Can Be Described as Half-Reactions
- Biological Oxidations Often Involve Dehydrogenation
- A Few Types of Coenzymes and Proteins Serve as Universal Electron Carriers
13.5 Regulation of Metabolic Pathways
- Cells and Organisms Maintain a Dynamic Steady State
- Both the Amount and the Catalytic Activity of an Enzyme Can Be Regulated
Chapter 14: Carbohydrate Metabolism Part 1: Glycolysis and Glycogen Synthesis
14.1 An Overview of Glycolysis
- Glycolysis Has Two Phases: The Preparatory and Payoff Phases
- In Glycolysis the Potential Energy of Glucose is Partially Converted to ATP and NADH
- Phosphorylated Intermediates are Important in Glycolysis
14.2 The Preparatory and Payoff Phases of Glycolysis
- The Preparatory Phase of Glycolysis Converts Glucose to a 3-carbon Metabolite and Consumes ATP
- The Payoff Phase of Glycolysis Yields ATP, NADH, and Pyruvate
- The Glycolytic Pathway Conserves Part of the Energy Released as ATP and NADH:
- Feeder Pathways Provide Additional Fuel for Glycolysis
14.3 Anaerobic Fermentation of Pyruvate
- There Are Two Anaerobic Fermentation Pathways
- The Warburg Effect Describes How Cancer Cells Rely Almost Entirely on Glycolysis for Energy
14.4 The Pentose Phosphate Pathway
- The Pentose Phosphate Pathway Generates NADPH and Essential Pentose Phosphates
- The Oxidative Phase Produces NADPH and Pentose Phosphates
- The Nonoxidative Phase Recycles Pentose Phosphates to Glucose 6-Phosphate, Fructose 6-Phosphate, and Glyceraldehyde 3-Phosphate
- NADPH Produced by the Pentose Phosphate Pathway Defends Cells from Reactive Oxygen Species
- Deficiencies in the Oxidative Phase of the Pentose Phosphate Pathway Have Serious Health Consequences
14.5 Glycogen Synthesis
- Glycogen Provides a Specialized Molecular Structure for Glucose Storage
- The Sugar Nucleotide UDP-Glucose Donates Glucose for Glycogen Synthesis
- Defects in Glycogen Synthesis have Important Medical Consequences
Chapter 15: Carbohydrate Metabolism Part 2: Gluconeogenesis and Glycogen Degradation
15.1 Gluconeogenesis
- Gluconeogenesis and Glycolysis Share Many But Not All Steps and Enzymes
- Glycolysis Enzymes are Bypassed at Three Steps in Gluconeogenesis
- Gluconeogenesis is Energetically Expensive and Essential
15.2 Coordinated Regulation of Glycolysis and Gluconeogenesis
- Hexokinase Isozymes Are Affected Differently by Their Product, Glucose 6-Phosphate
- Phosphofructokinase-1 and Fructose 1,6-Bisphosphatase Are Reciprocally Regulated
- Fructose 2,6-Bisphosphate Is a Potent Allosteric Regulator of PFK-1 and FBPase-1
15.3 Breakdown of Glycogen and Its Regulation
- Glycogen Breakdown Is Catalyzed by Glycogen Phosphorylase
- Glycogen Phosphorylase Is Regulated by Hormone-Stimulated Phosphorylation and by Allosteric Effectors
- Allosteric and Hormonal Signals Coordinate Carbohydrate Metabolism Throughout the Body
Chapter 16: Pyruvate Oxidation and the Citric Acid Cycle
16.1 Conversion of Pyruvate to Acetyl-CoA
- The Citric Acid Cycle Occurs in Mitochondria
- Pyruvate Is Oxidized by Pyruvate Dehydrogenase to Generate Acetyl-CoA, NADH, and CO2
- The Pyruvate Dehydrogenase Complex Promotes a Multi-stage Reaction Sequence
- Pyruvate Dehydrogenase is Subject to Regulation
16.2 The Citric Acid Cycle
- Citrate, the First Tricarboxylic Acid, Forms in Step 1
- A Citrate Hydroxyl Group Moves in Step 2
- Following the Formation of Isocitrate, Two Oxidative Decarboxylations that Form CO2 Occur with Different Mechanisms
- Succinyl-CoA Synthetase Promotes the Formation of Succinate and GTP in Step 5
- The Final Three Steps Convert Succinate to Oxaloacetate Via a Common Oxidative Path
- The Energy of Oxidation is Conserved in the Citric Acid Cycle
- The Concentration of Key Metabolites Regulates Flux Through the Citric Acid Cycle
16.3 The Citric Acid Cycle as a Metabolic Hub
- The Citric Acid Cycle Plays a Central Role in Catabolism and Anabolism
- A Variety of Reactions Replenish Citric Acid Cycle Intermediates or Supplement Cycle Products
16.4 The Citric Acid Cycle Affects Cell State and Disease State
- Changes in Cell State Can be Accompanied by Flux Through a Non-canonical Citric Acid Cycle
- Vitamin Deficiencies Result in Disease
- Amino Acid Substitutions in Isocitrate Dehydrogenase Facilitate Tumor Growth
Chapter 17: Lipid Catabolism and Anabolism
17.1 The Fed State: Digestion, Synthesis, and Storage of Fats
- Biosynthesis of Fatty Acids Requires Two Enzyme Complexes
- Fatty Acid Synthesis Is Tightly Regulated
- Free Fatty Acids Are Incorporated Into Glycerolipids
- Triacylglycerol Biosynthesis Is Regulated by Hormones
17.2 Synthesis and Transport of Cholesterol
- Cholesterol Is Made from Acetyl-CoA in Four Stages
- Cholesterol Has Several Fates
- Cholesterol and Other Lipids Are Carried as Lipoprotein Particles
- HDL and LDL Cholesterol Enter Cells through Receptor-Mediated Interactions
- Dysregulation of Cholesterol Can Lead to Cardiovascular Disease
17.3 The Fasted State: Fatty Acid Oxidation and Production of Ketone Bodies
- Lipid Catabolism Occurs In Fasted States
- Fatty Acid Oxidation Occurs In The Mitochondria
- Regulation of Fatty Acid Oxidation By Compartmentalization
- Ketone Body are Formed in the Liver and Exported to Other Tissues
- Ketone Bodies Are Overproduced in Diabetes and Starvation
Chapter 18: Amino Acid Catabolism and Anabolism
18.1 The Worldwide Nitrogen Web and its Many Interfaces With Living Systems
- The Global Nitrogen Web Makes Atmospheric Nitrogen Available to Cells
- Nitrogen is Converted to Ammonia by Enzymes of the Nitrogenase Complex
- Ammonia Is Incorporated into Biomolecules through Glutamate and Glutamine
- Amino Groups are Distributed Primarily via Transamination Facilitated by Pyridoxal Phosphate
- Ammonia Generated by Some Cellular Processes is Toxic to Animals
- A Few Amino Acids Play Special Roles in Nitrogen Metabolism
18.2 Disposal of Amino Groups via the Urea Cycle
- In Extrahepatic Tissues, Amino Groups are Incorporated into Glutamine for Transport to the Liver
- The Urea Cycle Disposes of Excess Amino Groups
- Connections Among Metabolic Pathways Reduce the Energetic Cost of Urea Synthesis
18.3 Amino Acid Catabolism and Anabolism
- Amino Acid Catabolism Produces Pyruvate, Acetyl-CoA, and Citric Acid Cycle intermediates
- Several Enzyme Cofactors Play Important Roles in Amino Acid Catabolism
- Some Genetic Deficiency Diseases are Linked to Amino Acid Catabolism
- Amino Acid Anabolism is often Not the Reverse of Amino Acid Catabolism
- Organisms Vary Greatly in Their Ability to Synthesize the 20 Common Amino Acids
- Ketoglutarate Gives Rise to Glutamate, Glutamine, Proline, and Arginine
18.4 Molecules Derived from Amino Acids
- Heme is Derived from Glycine and Succinyl-CoA
- Biological Amines Are Products of Amino Acid Decarboxylation
- Glutathione is Synthesized from Glutamate, Cysteine, and Glycine
18.5 Nucleotide Biosynthesis
- The Ribose in Nucleotides is Derived from Phosphoribosyl Pyrophosphate
- Pyrimidine Nucleotides Are Made from Aspartate, PRPP, and Carbamoyl Phosphate
- De Novo Purine Nucleotide Synthesis Begins with PRPP
- Ribonucleotides Are the Precursors of Deoxyribonucleotides
- Thymidylate Is Derived from dCDP and dUMP
Chapter 19: Electron Transfer and Oxidative Phosphorylation
19.1 The Mitochondrial Electron Transport Chain
- Chemiosmotic Theory Describes How Electron Flow Couples to ATP Synthesis in Mitochondria
- Mitochondrial Architecture Facilitates Electron Transport and ATP Synthesis
- Dehydrogenases Funnel Electrons to Universal Electron Acceptors
- Electrons Pass through a Series of Membrane-Bound Carriers
- Electron Carriers Function in Multienzyme Complexes
- The Energy of Electron Transfer is Conserved in a Proton Gradient
- Reactive Oxygen Species are Generated during Oxidative Phosphorylation
19.2 ATP Synthesis
- In the Chemiosmotic Model, Oxidation and Phosphorylation Are Obligately Coupled
- ATP Synthase Has Two Functional Domains
- Chemiosmotic Coupling Allows Nonintegral Stoichiometries of Consumption and ATP Synthesis
- Shuttle Systems Indirectly Convey Cytosolic NADH into Mitochondria for Oxidation
- Uncoupling the Proton Gradient from ATP Synthesis Produces Heat
19.3 Regulation of Oxidative Phosphorylation and Mitochondrial Disease
- An Inhibitory Protein Prevents ATP Hydrolysis during Hypoxia
- Hypoxia Leads to ROS Production and Several Adaptive Responses
- ATP Producing Pathways Are Regulated
- Mitochondrial Enzyme Defects Cause Disease
Chapter 20: Metabolism and Biosignaling
20.1 Hormone Structure and Action
- Hormones Act Through Specific High-Affinity Cellular Receptors
- Hormones are Chemically Diverse
- Hormones Regulate Glucose Levels
- Diabetes Mellitus Arises from Defects in Insulin Production or Action
20.2 Tissue Specific Metabolism
- The Liver Processes and Distributes Nutrients in Feeding
- The Liver Produces Ketone Bodies to Fuel Peripheral Tissues in Fasting
- Adipose Tissue Stores and Supplies Fatty Acids
- Muscle Uses ATP for Mechanical Work
20.3 Hormonal Regulation of Satiety and Body Weight
- Body Weight is Tightly Regulated by Hormones
- Adipose Tissue Produces Multiple Adipokines to Regulate Metabolism
- The Digestive System Regulates Satiety
Appendix A: Self-Check Answers
Appendix B: Section Review Questions and Answers
Appendix C: Chapter Review Questions and Answers
Product Updates
Authors
-
Michael Cox
Michael M. Cox was born in Wilmington, Delaware. In his first biochemistry course, the first edition of Lehninger's Biochemistry was a major influence in refocusing his fascination with biology and inspiring him to pursue a career in biochemistry. After graduate work at Brandeis University with William P. Jencks and post-doctoral work at Stanford with I. Robert Lehman, he moved to the University of Wisconsin–Madison in 1983. He became a full professor of Biochemistry in 1992.
Mike Cox has coordinated an active research team at Wisconsin investigating the function and mechanism of enzymes that act at the interface of DNA replication, repair, and recombination. That work has resulted in over 200 publications to date.
For more than three decades, Cox has taught introductory biochemistry to undergraduates and has lectured in a variety of graduate courses. He organized a course on professional responsibility for first-year graduate students and established a systematic program to draw talented biochemistry undergraduates into the laboratory at an early stage of their college career. He has received multiple awards for both his teaching and his research, including the Eli Lilly Award in Biological Chemistry, election as an AAAS fellow, and the UW Regents Teaching Excellence Award. Cox's hobbies include turning 18 acres of Wisconsin farmland into an arboretum, wine collecting, and assisting in the design of laboratory buildings.
-
Aaron Hoskins
Aaron A. Hoskins was born in Lafayette, Indiana, received his BS in chemistry from Purdue in 2000, and earned his PhD in biological chemistry at Massachusetts Institute of Technology with JoAnne Stubbe. In 2006, he went to Brandeis and University of Massachusetts Medical School as a postdoctoral fellow with Melissa Moore and Jeff Gelles. Hoskins joined the University of Wisconsin–Madison biochemistry faculty in 2011.
Hoskins's PhD research was on de novo purine biosynthesis. At Brandeis and University of Massachusetts, he began to study eukaryotic pre-mRNA splicing. During this time, he developed new single-molecule microscopy tools for studying the spliceosome.
Hoskins's laboratory is focused on understanding how spliceosomes are assembled and regulated and how they recognize introns. Hoskins has won awards for his research, including being named a Beckman Young Investigator and Shaw Scientist. He has taught introductory biochemistry for undergraduates since 2012. Hoskins also enjoys playing with his cat (Louise) and dog (Agatha), yoga/exercise, and tries to read a new book each week.
-
Alain Viel
Alain Viel a native of Vesoul (France), earned his PhD in developmental Biology from the University of Paris VI (France), studying under the guidance of Prof. Hermann Denis and Marc le Maire. His research focused on the storage of proteins and RNAs during amphibian oogenesis and using biochemical approaches. As a postdoctoral fellow in Prof. Daniel Branton's lab at Harvard University, he made significant strides in understanding the structure-function relationship of spectrin and the role of spliced variants of the tumor suppressor Hdlg.
In 2004, Viel became a senior lecturer in the Department of Molecular and Cellular Biology at Harvard University. He created LS100, a course where Harvard undergraduate students experience how science is done through the practice of experimental inquiry. His interest in developing accessible teaching material prompted Viel to create a blended-learning biochemistry course (MCB63) concomitantly with a version of the course for HarvardX (MCB63X). He has also developed an online course introducing non-scientists to CRISPR and its application in the life sciences.
Viel is the faculty co-director of LabXchange, an educational platform created at Harvard University. LabXchange offers an integrated teaching and learning ecosystem, engages with a diverse, global community, and distributes free high-quality STEM educational material, allowing everyone, everywhere, to chart a path in science.
Viel's influence extends beyond the classroom. As a founding member of BioVisions, a collaboration between scientists, teaching faculty, students, and media professionals, he has contributed to science visualization on a global scale. His collaborations with Prof. Lue have resulted in award-winning animations depicting biological processes in the context of the cell. These animations are widely used in high schools, colleges, museums, and TV series across the United States and Europe.
Viel was awarded the UNESCO Chair on Life Sciences and Social Innovation in 2023 for his contributions to digital education.
-
Judith Simcox
Judith Simcox grew up in Montana, earning her PhD in Biochemistry from the University of Utah studying with Dr. Donald McClain. As a graduate student, her research focused on circadian regulation of glucose metabolism. She then moved to studying the regulation of energy expenditure by lipid processing in her postdoctoral fellowship in the lab of Dr. Claudio Villanueva.
In 2019, Simcox became an Associate Professor of Biochemistry at the University of Wisconsin-Madison exploring inter-organ communication through lipid derived metabolites. By focusing on the lipids in the blood plasma, the Simcox lab asks the basic questions of: what tissues are producing these lipids and what is their function? The goal of this work is to understand how these lipids are functioning to drive metabolic diseases including cardiovascular disease, type 2 diabetes, and Alzheimer's disease. This work has won Simcox several awards including the 2023 American Society for Biochemistry and Molecular Biology (ASBMB) Walter A Shaw Young Investigator Award in Lipid Research, 2022 Journal of Lipid Research (JLR) Rising Star in Lipid Research Award, and selection as a Howard Hughes Medical Institute Freeman Hrabowski Scholar.
Simcox teaches biochemistry for undergraduates since 2019, focusing on modules to explore metabolism. She is a nationally recognized leader in building programs to increase access to science for her work with the Native American Research Internship at the University of Utah, and the Center for Indigenous Research to Create Learning and Excellence at the University of Wisconsin-Madison. In her free time, Simcox enjoys walks with her dogs (Roo, Ginger, Barney), bird watching, and running.
Table of Contents
Chapter 1: Biochemistry Concepts and Themes
1.1 Science and the Scientific Method
- What is Science?
- What is the Scientific Method?
1.2 Organisms, Cells, Chromosomes, and Genes
- Organisms Belong to Three Distinct Domains of Life
- Cells Are the Structural and Functional Units of All Living Organisms
- Viruses Cannot Live Independently of Cells
- Bacterial Cells Feature a Relatively Simple Architecture and Streamlined Lifestyles
- Eukaryotic Cells Have a Variety of Membranous Organelles
- Cells Contain a Wide Range of Supramolecular Structures
- Major Model Organisms and Systems are Useful in Biochemistry
- The Linear Sequence in DNA Encodes Proteins with Three-Dimensional Structures
1.3 The Organic Chemistry of Biochemistry
- Major Organic Species are Found in Cells
- Macromolecules Are the Major Constituents of Cells
- Molecular Weight and Molecular Mass are Expressed by Distinct Conventions
- Nucleophiles and Electrophiles Define How Many Reactions Proceed
- Cofactors Facilitate Particular Classes of Biochemical Reactions
1.4 A Review of Basic Thermodynamics
- Equilibrium Constants and Rate Constants Describe Distinct but Related Thermodynamic Parameters
- Organisms Transform Energy and Matter from Their Surroundings
- Creating and Maintaining Order Requires Work and Energy
1.5 Using Data Banks
Chapter 2: Water: The Chemistry of Life
2.1 Weak Interactions in Aqueous Systems
- Hydrogen Bonds Give Water Its Unusual Properties
- Water Interacts Electrostatically with Charged Solutes
- Nonpolar Gases Are Poorly Soluble in Water
- The Hydrophobic Effect is an Entropy-based Phenomenon
- van der Waals Interactions and Other Weak Interactions Are Key to Macromolecular Structure and Function
2.2 Ionization of Water, Weak Acids, and Weak Bases
- The Ionization of Water Is Expressed by an Equilibrium Constant
- The pH Scale Designates H+ and OH– Concentrations
- Weak Acids and Bases Have Characteristic Acid Dissociation Constants
- Titration Curves Reveal the pKa of Weak Acids
2.3 Buffering against pH Changes in Biological Systems
- A Buffer System Resists Changes in pH in Response to Added Acid or Base.
- The Henderson-Hasselbalch Equation Relates pH, pKa, and Buffer Concentration
- Weak Acids or Bases Buffer Cells and Tissues against pH Changes
- Phosphate and Bicarbonate Are Important Biological Buffer Systems Untreated Diabetes Produces Life-Threatening Acidosis
Chapter 3: Amino Acids, Peptides, and Proteins
3.1 Amino Acids
- What is an Amino Acid?
- The Amino Acid Residues in Proteins Are L Stereoisomers
- Amino Acids Can Be Classified by R Group
- Some Amino Acids Absorb Ultraviolet Light
- Uncommon Amino Acids Also Have Important Functions
- Amino Acids Can Act as Acids and Bases
- Amino Acids Differ in Their Acid-Base Properties
3.2 Peptides and Proteins
- Peptides Are Chains of Amino Acids
- Disulfide Bonds Occur in Some Proteins
- Ionization Behavior Can Distinguish Peptides
- Some Proteins Contain Chemical Groups Other Than Amino Acids
3.3 Purifying Proteins
- Proteins Can Be Separated and Purified
- Proteins Are Detected and Quantified Based on Their Functions
- Proteins Can Be Separated and Characterized by Electrophoresis
3.4 The Primary Structure of Proteins and Protein Chemistry
- There are Levels of Complexity to Protein Structure
- The Function of a Protein Depends on Its Amino Acid Sequence
- There are Multiple Ways to Reduce a Polypeptide Chain into Fragments.
- Mass Spectrometry Provides Information on Molecular Mass, Amino Acid Sequence, and Entire Proteomes
- Amino Acid Sequences Provide Important Biochemical and Evolutionary Information
Chapter 4: Protein Structure
4.1 Forces and Interactions that Stabilize Protein Structures
- Protein Structures Are Largely Stabilized by Weak Interactions
- Hydrogen Bonding, Ion Pairs, and van der Waals Interactions Also Contribute to Protein Folding
- The Conformation of the Peptide Bond Constrains Polypeptide Conformation
4.2 Secondary Protein Structure
- The α Helix Maximizes the Use of Polypeptide Hydrogen Bonds
- The β Strand is a Common Secondary Structure with an Extended Conformation
- Ramachandran Plots Describe the Distribution of Secondary Structure in a Protein
4.3 Tertiary and Quaternary Protein Structure
- Fibrous Proteins Have a Single Type of Secondary Structure
- The Fibrous Protein Collagen is the Most Abundant Protein in Mammals
- Silk is Made from a Fibrous Protein with b-sheet Secondary Structure
- Globular Proteins are Compact and Highly Varied in Three Dimensional Structure
- Protein Tertiary Structures can be Described in Terms of Motifs and Domains.
- Intrinsically Disordered Proteins Lack Stable Tertiary Structures.
- Quaternary Structure Describes the Organization of Multisubunit Proteins.
- Biomolecular Structures Can be Determined Using a Variety of Methods
- The Protein Data Bank is a Repository for Biomolecular Structures
4.4 Protein Denaturation and Folding
- Loss of Protein Structure Results in Loss of Function
- Amino Acid Sequence Determines Tertiary Structure
- Protein Folding Occurs by Defined Pathways and can be Assisted by Chaperones.
- Defects in Protein Folding Cause Human Disease
Chapter 5: Protein Function and Ligand Binding
5.1 Reversible Protein-Ligand Binding
- Ligands Bind to Proteins Reversibly at Binding Sites
- Protein-Ligand Interactions Can Be Described Quantitatively
5.2 Reversible Binding of a Protein to a Ligand: Oxygen-Binding by Myoglobin
- Oxygen Can Bind to a Heme Prosthetic Group
- Globins Are a Family of Oxygen-Binding Proteins
- The Binding of Oxygen to Myoglobin can be Described Quantitatively
- Protein Structure Affects How Ligands Bind
5.3 Reversible and Cooperative Binding of a Protein to a Ligand: Oxygen-Binding by Hemoglobin
- Hemoglobin Subunits Are Structurally Similar to Myoglobin
- Hemoglobin Undergoes a Structural Change on Binding Oxygen
- Hemoglobin Binds Oxygen Cooperatively
- Cooperative Ligand Binding Can Be Described Quantitatively
- Hemoglobin Also Transports H+ and CO2
5.4 Medical Conditions Related to Hemoglobin
- CO Binding to Hemoglobin Poses a Serious Health Risk
- Altered Hemoglobin Subunit Interactions in Sickle Cell Anemia Cause Pain and Suffering
Chapter 6: Protein Function and Enzymes
6.1 What are Enzymes?
- Most Enzymes Are Proteins
- Enzyme-catalyzed Reactions Occur Within Active Sites
- Enzymes Affect Reaction Rates, Not Equilibria
- Reaction Rates and Equilibria are Described by Constants
6.2 How Enzymes Work
- Noncovalent Interactions between Enzyme and Substrate Are Optimized in the Transition State
- Enzymes Use a Variety of Additional Chemical Mechanisms to Facilitate Catalysis
- Coenzymes Facilitate Particular Types of Reactions
6.3 Enzyme Kinetics
- The Steady State of an Enzyme-catalyzed Reaction Reflects the Concentration of ES
- The Relationship Between Substrate Concentration and Reaction Rate can be Described Quantitatively
- Scientists Compare Enzymes Using Vmax and Km.
- Enzymes are Subject to Reversible and Irreversible Inhibition
6.4 Chymotrypsin and Enzymatic Catalysis
- The Chymotrypsin Mechanism Involves Acylation and Deacylation of an Active Site Ser Residue
- An Understanding of Protease Mechanisms Led to Treatments for HIV
- An Understanding of Enzyme Mechanism Leads to Useful Antibiotics
6.5 Regulatory Enzymes
- Some Enzymes are Regulated by Allosteric Conformational Changes in Response to Modulator Binding
- Some Enzymes are Regulated by Reversible Covalent Modification
- Some Enzymes are Regulated by Proteolytic Cleavage of an Enzyme Precursor
Chapter 7: Carbohydrates
7.1 Monosaccharides and Disaccharides
- The Two Families of Monosaccharides Are Aldoses and Ketoses
- The Common Monosaccharides Have Cyclic Structures
- Sugars Containing and Forming Aldehydes are Reducing Sugars
- Disaccharides Consist of Two Monosaccharides Joined by a Glycosidic Bond
7.2 Polysaccharides
- Some Homopolysaccharides Are Storage Forms of Fuel While Others have Structural Roles
- Glycosaminoglycans Are Heteropolysaccharides of the Extracellular Matrix
7.3 Glycoconjugates: Peptidoglycans, Proteoglycans, Glycoproteins, and Glycolipids
- Peptidoglycan Reinforces the Bacterial Cell Wall
- Proteoglycans Are Glycosaminoglycan-Containing Macromolecules of the Cell Surface and Extracellular Matrix
- Glycoproteins Are Proteins with Covalently Attached Oligosaccharides
- Glycolipids and Lipopolysaccharides Are Membrane Components
7.4 Carbohydrates as Signaling Molecules
- Oligosaccharides Have Highly Diverse Structures
- Lectins Are Proteins That Bind Specifically to Complex Oligosaccharides and Mediate Many Biological Processes
Chapter 8: Lipids, Membranes, and Membrane Proteins
8.1 Membrane Lipids
- Fatty Acids are the Hydrocarbon Chain of Membrane Lipids
- Fatty Acid Composition of Lipids Impacts Health
- Structural Elements Determine Membrane Classes
- Membranes Lipids are Amphipathic Molecules that Form Lipid Bilayers
- Membrane Lipid Composition Impacts Membrane Fluidity
8.2 The Architecture of Membrane Proteins
- Membrane Proteins Differ in How They Associate with the Membrane Bilayer
- Integral Membrane Proteins Span Membranes and Can be Transporters
- Peripheral Membrane Proteins Interact with Membranes through Electrostatic Charge
- Lipid-anchored Proteins are Covalently Linked to Hydrophobic Anchors Embedded in the Membrane
8.3 Moving Molecules Through Membranes
- Membrane Transporters are Required to Move Large and Charged Molecules across Membranes
- Transport in and out of Cells May be Passive or Active
- Transporters and Ion Channels Share Structural Properties but Have Different Mechanisms
- The Glucose Transporter of Erythrocytes Mediates Passive Transport
- P-Type ATPases are Active Transporters that Change Conformation with Phosphoryl- Group Transfer from ATP
- Ion Channels Allow Rapid Movement of Ions Across Membranes
Chapter 9: Nucleotides and Nucleic Acids
9.1 Nucleotides
- Nucleotides Have Three Molecular Components
- The Common Nucleotides Have Many Uncommon Variants
- Phosphodiester Bonds Link Successive Nucleotides in Nucleic Acids
- The Properties of Nucleotide Bases Affect the Three-Dimensional Structure of Nucleic Acids
9.2 Nucleic Acid Structures
- DNA Is a Double Helix That Stores Genetic Information
- DNA Can Occur in Different Three-Dimensional Forms
- Certain DNA and RNA Sequences Adopt Unusual Structures
- Messenger RNAs Code for Polypeptide Chains
- Many RNAs Have More Complex Three-Dimensional Structures
9.3 Nucleic Acid Chemistry
- Double-Helical DNA and RNA Can Be Denatured
- Base Stacking Affects the UV Absorption Properties of DNA and RNA
- Nucleotides and Nucleic Acids Undergo Nonenzymatic Transformations
9.4 Nucleotide Roles in Cell Energetics and Signaling
- Nucleotides Carry Chemical Energy in Cells
- Some Nucleotides Are Regulatory Molecules or Signals
- Adenine Nucleotides Serve as Constituents of Many Enzymatic Cofactors; a Clue to the Origin of Life?
Chapter 10: Biological Information Part 1: DNA and RNA Metabolism
10.1 DNA Replication
- DNA Replication Follows a Set of Rules
- DNA Polymerases Synthesizes DNA
- DNA Replication Requires Many Enzymes and Protein Factors
- DNA Replication Occurs in Stages
10.2 DNA Repair and Organization
- All Cells Have Multiple DNA Repair Systems
- DNA Repair Can Also Occur in the Absence of Replication
- DNA Is Organized into Chromatin
10.3 Transcription and RNA Processing
- RNA Polymerases Synthesizes RNA
- RNA Replication Requires Many Enzymes and Protein Factors
- RNA Syntheses Occurs in Stages
- Medicines can Target or Be Made by RNA Polymerases
- Nearly All Eukaryotic RNAs Must Be Processed
- Reverse Transcriptases Produce DNA From RNA
10.4 Regulation of Transcription
- Transcription of Specific Genes Requires Regulatory Proteins in Addition to RNA Polymerase
- Regulation of Gene Expression in Bacteria
- Regulation of Gene Expression in Eukaryotes
Chapter 11: Biological Information Part 2: Protein Metabolism
11.1 The Genetic Code
- The Genetic Code Describes How Sets of Nucleic Acids Correspond to Particular Amino Acids
- tRNA Anticodons Base Pair with Codons
- tRNAs are Charged with Amino Acids for Protein Synthesis
- tRNA Charging Requires ATP Hydrolysis
11.2 Structure and Function of Ribosomes
- Ribosomes Catalyze Protein Synthesis
- Protein Synthesis Occurs in Stages
- Translation Factors Interact with the Ribosome During Elongation and Termination
- Protein Synthesis by the Ribosomes is Energetically Expensive
11.3 Protein Folding, Modification, and Degradation
- Chaperones Help Proteins Fold into Their Native Conformation
- Posttranslational Modifications are Critical for the Function of Many Proteins
- Protein Degradation is Highly Regulated in Eukaryotes by the Ubiquitin/Proteosome Pathway
11.4 Translational Control
- Riboswitches, Small RNAs, and Attenuation Can Control Gene Expression in Bacteria
- Eukaryotes Use mRNA Binding Proteins, RNAi, and MicroRNAs to Regulate Protein Production
Chapter 12: Nucleic Acid Technologies
12.1 Defining Genomic Information
- The Genome is All of the Nucleic Acid Needed to Support the Life of an Organism
- The Polymerase Chain Reaction Provides Targeted Amplification of Genomic Information
- DNA Can Be Sequenced
- Sanger Sequencing has been Automated
- Next-Generation DNA Sequencing Produces Complete Genome Sequences
- RNA Can be Sequenced by First Copying the RNA to DNA with Reverse Transcriptase
12.2 Altering Genomic Information
- Joining DNA Segments from Different Sources Yields Recombinant DNA Segments Can be Joined Without Using Restriction Enzymes
- Cloned DNA Can be Altered to Study Genes and Proteins
- CRISPR/Cas Systems Allows Targeted Cleavage or Modification of Genomic Information
12.3 Using Genomic Information
- An Altered Genome can Lead to an Altered Transcriptome and Proteome
- Genomic Information Can be Used to Identify the Source of Genetic Diseases
- Genomic Information Can be Used to Investigate Crimes
Chapter 13: Introduction to Intermediary Metabolism
13.1 What is Metabolism?
- Molecules are Metabolized by Anabolic and Catabolic Pathways
- Metabolic Pathways can be Converging, Diverging, or Cyclic
13.2 Common Enzyme Reactions in Metabolism
- Carbonyls are Important for Making and Breaking Carbon–Carbon Bonds
- Rearrangement and Isomerization Reactions Reposition Reactive Groups
- Elimination Reactions Release Good Leaving Groups
- Free-Radical Reactions Involve Complex Rearrangements
- Group Transfer Reactions Add or Subtract Functional Groups to Metabolites
- Oxidation-Reduction Reactions Involve Electron Transfer to or from Biomolecules
13.3 ATP and Phosphoryl Group Transfers
- ATP Contains High Energy Phosphodiester Bonds
- ATP Hydrolysis is Thermodynamically Very Favorable
- Many Other Metabolites and Enzyme Reaction Intermediates Also Have Large, Negative Free Energies of Hydrolysis
- ATP Donates Phosphoryl, Pyrophosphoryl, and Adenyl Groups
- ATP can Provide Energy by Group Transfers, Not Just by Hydrolysis
13.4 Biological Oxidation-Reduction Reactions
- Oxidation-Reduction Reactions Can Be Described as Half-Reactions
- Biological Oxidations Often Involve Dehydrogenation
- A Few Types of Coenzymes and Proteins Serve as Universal Electron Carriers
13.5 Regulation of Metabolic Pathways
- Cells and Organisms Maintain a Dynamic Steady State
- Both the Amount and the Catalytic Activity of an Enzyme Can Be Regulated
Chapter 14: Carbohydrate Metabolism Part 1: Glycolysis and Glycogen Synthesis
14.1 An Overview of Glycolysis
- Glycolysis Has Two Phases: The Preparatory and Payoff Phases
- In Glycolysis the Potential Energy of Glucose is Partially Converted to ATP and NADH
- Phosphorylated Intermediates are Important in Glycolysis
14.2 The Preparatory and Payoff Phases of Glycolysis
- The Preparatory Phase of Glycolysis Converts Glucose to a 3-carbon Metabolite and Consumes ATP
- The Payoff Phase of Glycolysis Yields ATP, NADH, and Pyruvate
- The Glycolytic Pathway Conserves Part of the Energy Released as ATP and NADH:
- Feeder Pathways Provide Additional Fuel for Glycolysis
14.3 Anaerobic Fermentation of Pyruvate
- There Are Two Anaerobic Fermentation Pathways
- The Warburg Effect Describes How Cancer Cells Rely Almost Entirely on Glycolysis for Energy
14.4 The Pentose Phosphate Pathway
- The Pentose Phosphate Pathway Generates NADPH and Essential Pentose Phosphates
- The Oxidative Phase Produces NADPH and Pentose Phosphates
- The Nonoxidative Phase Recycles Pentose Phosphates to Glucose 6-Phosphate, Fructose 6-Phosphate, and Glyceraldehyde 3-Phosphate
- NADPH Produced by the Pentose Phosphate Pathway Defends Cells from Reactive Oxygen Species
- Deficiencies in the Oxidative Phase of the Pentose Phosphate Pathway Have Serious Health Consequences
14.5 Glycogen Synthesis
- Glycogen Provides a Specialized Molecular Structure for Glucose Storage
- The Sugar Nucleotide UDP-Glucose Donates Glucose for Glycogen Synthesis
- Defects in Glycogen Synthesis have Important Medical Consequences
Chapter 15: Carbohydrate Metabolism Part 2: Gluconeogenesis and Glycogen Degradation
15.1 Gluconeogenesis
- Gluconeogenesis and Glycolysis Share Many But Not All Steps and Enzymes
- Glycolysis Enzymes are Bypassed at Three Steps in Gluconeogenesis
- Gluconeogenesis is Energetically Expensive and Essential
15.2 Coordinated Regulation of Glycolysis and Gluconeogenesis
- Hexokinase Isozymes Are Affected Differently by Their Product, Glucose 6-Phosphate
- Phosphofructokinase-1 and Fructose 1,6-Bisphosphatase Are Reciprocally Regulated
- Fructose 2,6-Bisphosphate Is a Potent Allosteric Regulator of PFK-1 and FBPase-1
15.3 Breakdown of Glycogen and Its Regulation
- Glycogen Breakdown Is Catalyzed by Glycogen Phosphorylase
- Glycogen Phosphorylase Is Regulated by Hormone-Stimulated Phosphorylation and by Allosteric Effectors
- Allosteric and Hormonal Signals Coordinate Carbohydrate Metabolism Throughout the Body
Chapter 16: Pyruvate Oxidation and the Citric Acid Cycle
16.1 Conversion of Pyruvate to Acetyl-CoA
- The Citric Acid Cycle Occurs in Mitochondria
- Pyruvate Is Oxidized by Pyruvate Dehydrogenase to Generate Acetyl-CoA, NADH, and CO2
- The Pyruvate Dehydrogenase Complex Promotes a Multi-stage Reaction Sequence
- Pyruvate Dehydrogenase is Subject to Regulation
16.2 The Citric Acid Cycle
- Citrate, the First Tricarboxylic Acid, Forms in Step 1
- A Citrate Hydroxyl Group Moves in Step 2
- Following the Formation of Isocitrate, Two Oxidative Decarboxylations that Form CO2 Occur with Different Mechanisms
- Succinyl-CoA Synthetase Promotes the Formation of Succinate and GTP in Step 5
- The Final Three Steps Convert Succinate to Oxaloacetate Via a Common Oxidative Path
- The Energy of Oxidation is Conserved in the Citric Acid Cycle
- The Concentration of Key Metabolites Regulates Flux Through the Citric Acid Cycle
16.3 The Citric Acid Cycle as a Metabolic Hub
- The Citric Acid Cycle Plays a Central Role in Catabolism and Anabolism
- A Variety of Reactions Replenish Citric Acid Cycle Intermediates or Supplement Cycle Products
16.4 The Citric Acid Cycle Affects Cell State and Disease State
- Changes in Cell State Can be Accompanied by Flux Through a Non-canonical Citric Acid Cycle
- Vitamin Deficiencies Result in Disease
- Amino Acid Substitutions in Isocitrate Dehydrogenase Facilitate Tumor Growth
Chapter 17: Lipid Catabolism and Anabolism
17.1 The Fed State: Digestion, Synthesis, and Storage of Fats
- Biosynthesis of Fatty Acids Requires Two Enzyme Complexes
- Fatty Acid Synthesis Is Tightly Regulated
- Free Fatty Acids Are Incorporated Into Glycerolipids
- Triacylglycerol Biosynthesis Is Regulated by Hormones
17.2 Synthesis and Transport of Cholesterol
- Cholesterol Is Made from Acetyl-CoA in Four Stages
- Cholesterol Has Several Fates
- Cholesterol and Other Lipids Are Carried as Lipoprotein Particles
- HDL and LDL Cholesterol Enter Cells through Receptor-Mediated Interactions
- Dysregulation of Cholesterol Can Lead to Cardiovascular Disease
17.3 The Fasted State: Fatty Acid Oxidation and Production of Ketone Bodies
- Lipid Catabolism Occurs In Fasted States
- Fatty Acid Oxidation Occurs In The Mitochondria
- Regulation of Fatty Acid Oxidation By Compartmentalization
- Ketone Body are Formed in the Liver and Exported to Other Tissues
- Ketone Bodies Are Overproduced in Diabetes and Starvation
Chapter 18: Amino Acid Catabolism and Anabolism
18.1 The Worldwide Nitrogen Web and its Many Interfaces With Living Systems
- The Global Nitrogen Web Makes Atmospheric Nitrogen Available to Cells
- Nitrogen is Converted to Ammonia by Enzymes of the Nitrogenase Complex
- Ammonia Is Incorporated into Biomolecules through Glutamate and Glutamine
- Amino Groups are Distributed Primarily via Transamination Facilitated by Pyridoxal Phosphate
- Ammonia Generated by Some Cellular Processes is Toxic to Animals
- A Few Amino Acids Play Special Roles in Nitrogen Metabolism
18.2 Disposal of Amino Groups via the Urea Cycle
- In Extrahepatic Tissues, Amino Groups are Incorporated into Glutamine for Transport to the Liver
- The Urea Cycle Disposes of Excess Amino Groups
- Connections Among Metabolic Pathways Reduce the Energetic Cost of Urea Synthesis
18.3 Amino Acid Catabolism and Anabolism
- Amino Acid Catabolism Produces Pyruvate, Acetyl-CoA, and Citric Acid Cycle intermediates
- Several Enzyme Cofactors Play Important Roles in Amino Acid Catabolism
- Some Genetic Deficiency Diseases are Linked to Amino Acid Catabolism
- Amino Acid Anabolism is often Not the Reverse of Amino Acid Catabolism
- Organisms Vary Greatly in Their Ability to Synthesize the 20 Common Amino Acids
- Ketoglutarate Gives Rise to Glutamate, Glutamine, Proline, and Arginine
18.4 Molecules Derived from Amino Acids
- Heme is Derived from Glycine and Succinyl-CoA
- Biological Amines Are Products of Amino Acid Decarboxylation
- Glutathione is Synthesized from Glutamate, Cysteine, and Glycine
18.5 Nucleotide Biosynthesis
- The Ribose in Nucleotides is Derived from Phosphoribosyl Pyrophosphate
- Pyrimidine Nucleotides Are Made from Aspartate, PRPP, and Carbamoyl Phosphate
- De Novo Purine Nucleotide Synthesis Begins with PRPP
- Ribonucleotides Are the Precursors of Deoxyribonucleotides
- Thymidylate Is Derived from dCDP and dUMP
Chapter 19: Electron Transfer and Oxidative Phosphorylation
19.1 The Mitochondrial Electron Transport Chain
- Chemiosmotic Theory Describes How Electron Flow Couples to ATP Synthesis in Mitochondria
- Mitochondrial Architecture Facilitates Electron Transport and ATP Synthesis
- Dehydrogenases Funnel Electrons to Universal Electron Acceptors
- Electrons Pass through a Series of Membrane-Bound Carriers
- Electron Carriers Function in Multienzyme Complexes
- The Energy of Electron Transfer is Conserved in a Proton Gradient
- Reactive Oxygen Species are Generated during Oxidative Phosphorylation
19.2 ATP Synthesis
- In the Chemiosmotic Model, Oxidation and Phosphorylation Are Obligately Coupled
- ATP Synthase Has Two Functional Domains
- Chemiosmotic Coupling Allows Nonintegral Stoichiometries of Consumption and ATP Synthesis
- Shuttle Systems Indirectly Convey Cytosolic NADH into Mitochondria for Oxidation
- Uncoupling the Proton Gradient from ATP Synthesis Produces Heat
19.3 Regulation of Oxidative Phosphorylation and Mitochondrial Disease
- An Inhibitory Protein Prevents ATP Hydrolysis during Hypoxia
- Hypoxia Leads to ROS Production and Several Adaptive Responses
- ATP Producing Pathways Are Regulated
- Mitochondrial Enzyme Defects Cause Disease
Chapter 20: Metabolism and Biosignaling
20.1 Hormone Structure and Action
- Hormones Act Through Specific High-Affinity Cellular Receptors
- Hormones are Chemically Diverse
- Hormones Regulate Glucose Levels
- Diabetes Mellitus Arises from Defects in Insulin Production or Action
20.2 Tissue Specific Metabolism
- The Liver Processes and Distributes Nutrients in Feeding
- The Liver Produces Ketone Bodies to Fuel Peripheral Tissues in Fasting
- Adipose Tissue Stores and Supplies Fatty Acids
- Muscle Uses ATP for Mechanical Work
20.3 Hormonal Regulation of Satiety and Body Weight
- Body Weight is Tightly Regulated by Hormones
- Adipose Tissue Produces Multiple Adipokines to Regulate Metabolism
- The Digestive System Regulates Satiety
Appendix A: Self-Check Answers
Appendix B: Section Review Questions and Answers
Appendix C: Chapter Review Questions and Answers
Product Updates
The core of biochemistry in Macmillan Learning’s acclaimed Achieve
Achieve for Lehninger Biochemistry: Core Concepts and Applications is a breakthrough interactive approach to mastering, practicing, and thinking critically about core biochemistry concepts. Created by the authors of the gold standard Lehninger Principles of Biochemistry, for chemistry, biophysics, and other STEM majors, it enhances coverage of the basics of biochemistry with relevant medical applications, interactive figures, scaffolded assessment, a Study Guide, adaptive quizzing, activities, and more.Success Stories
Here are a few examples of how Achieve has helped instructors like you improve student preparedness, enhance their sense of belonging, and achieve course goals they set for themselves.
Prof. Kiandra Johnson, Spelman College
See how the resources in Achieve help you engage students before, during, and after class.
Prof. Jennifer Duncan
Use diagnostics in Achieve for a snapshot into cognitive and non-cognitive factors that may impact your students’ preparedness.
Prof. Ryan Elsenpeter
Here’s why educators who use Achieve would recommend it to their peers.
Looking for instructor resources like Test Banks, Lecture Slides, and Clicker Questions? Request access to Achieve to explore the full suite of instructor resources.
FAQs
-
-
Are you a campus bookstore looking for ordering information?
MPS Order Search Tool (MOST) is a web-based purchase order tracking program that allows customers to view and track their purchases. No registration or special codes needed! Just enter your BILL-TO ACCT # and your ZIP CODE to track orders.
Canadian Stores: Please use only the first five digits/letters in your zip code on MOST.
Visit MOST, our online ordering system for booksellers: https://tracking.mpsvirginia.com/Login.aspx
Learn more about our Bookstore programs here: https://www.macmillanlearning.com/college/us/contact-us/booksellers
-
-
-
Our courses currently integrate with Canvas, Blackboard (Learn and Ultra), Brightspace, D2L, and Moodle. Click on the support documentation below to find out more details about the integration with each LMS.
Integrate Macmillan courses with Blackboard
Integrate Macmillan courses with Canvas
-
-
-
If you’re a verified instructor, you can request a free sample of our courseware, e-book, or print textbook to consider for use in your courses. Only registered and verified instructors can receive free print and digital samples, and they should not be sold to bookstores or book resellers. If you don't yet have an existing account with Macmillan Learning, it can take up to two business days to verify your status as an instructor. You can request a free sample from the right side of this product page by clicking on the "Request Instructor Sample" button or by contacting your rep. Learn more.
-
-
-
Sometimes also referred to as a spiral-bound or binder-ready textbook, loose-leaf textbooks are available to purchase. This three-hole punched, unbound version of the book costs less than a hardcover or paperback book.
-
-
-
Achieve (full course) includes our complete e-book, as well as online quizzing tools, multimedia assets, and iClicker active classroom manager.
Most Achieve Essentials courses do not include our e-books and adaptive quizzing.
Visit our comparison table for details: https://www.macmillanlearning.com/college/us/digital/achieve/compare
-
-
-
Achieve (full course) includes our complete e-book, as well as online quizzing tools, multimedia assets, and iClicker active classroom manager.
Achieve Read & Practice only includes our e-book and adaptive quizzing, and does not include instructor resources and assignable assessments. Read & Practice does integrate with LMS.
Visit our comparison table for details: https://www.macmillanlearning.com/college/us/digital/achieve/compare
-
-
-
We can help! Contact your representative to discuss your specific needs for your course. If our off-the-shelf course materials don’t quite hit the mark, we also offer custom solutions made to fit your needs.
-
ISBN:9781319434625
Access all your course tools in one place!
ISBN:9781319593988
Save money with our hole-punched, loose-leaf textbook.
ISBN:9781319605858
This package includes Achieve and Loose-Leaf.
FAQs
-
-
Are you a campus bookstore looking for ordering information?
MPS Order Search Tool (MOST) is a web-based purchase order tracking program that allows customers to view and track their purchases. No registration or special codes needed! Just enter your BILL-TO ACCT # and your ZIP CODE to track orders.
Canadian Stores: Please use only the first five digits/letters in your zip code on MOST.
Visit MOST, our online ordering system for booksellers: https://tracking.mpsvirginia.com/Login.aspx
Learn more about our Bookstore programs here: https://www.macmillanlearning.com/college/us/contact-us/booksellers
-
-
-
Our courses currently integrate with Canvas, Blackboard (Learn and Ultra), Brightspace, D2L, and Moodle. Click on the support documentation below to find out more details about the integration with each LMS.
Integrate Macmillan courses with Blackboard
Integrate Macmillan courses with Canvas
-
-
-
If you’re a verified instructor, you can request a free sample of our courseware, e-book, or print textbook to consider for use in your courses. Only registered and verified instructors can receive free print and digital samples, and they should not be sold to bookstores or book resellers. If you don't yet have an existing account with Macmillan Learning, it can take up to two business days to verify your status as an instructor. You can request a free sample from the right side of this product page by clicking on the "Request Instructor Sample" button or by contacting your rep. Learn more.
-
-
-
Sometimes also referred to as a spiral-bound or binder-ready textbook, loose-leaf textbooks are available to purchase. This three-hole punched, unbound version of the book costs less than a hardcover or paperback book.
-
-
-
Achieve (full course) includes our complete e-book, as well as online quizzing tools, multimedia assets, and iClicker active classroom manager.
Most Achieve Essentials courses do not include our e-books and adaptive quizzing.
Visit our comparison table for details: https://www.macmillanlearning.com/college/us/digital/achieve/compare
-
-
-
Achieve (full course) includes our complete e-book, as well as online quizzing tools, multimedia assets, and iClicker active classroom manager.
Achieve Read & Practice only includes our e-book and adaptive quizzing, and does not include instructor resources and assignable assessments. Read & Practice does integrate with LMS.
Visit our comparison table for details: https://www.macmillanlearning.com/college/us/digital/achieve/compare
-
-
-
We can help! Contact your representative to discuss your specific needs for your course. If our off-the-shelf course materials don’t quite hit the mark, we also offer custom solutions made to fit your needs.
-
Lehninger Biochemistry: Core Concepts and Applications
Achieve for Lehninger Biochemistry: Core Concepts and Applications is a breakthrough interactive approach to mastering, practicing, and thinking critically about core biochemistry concepts. Created by the authors of the gold standard Lehninger Principles of Biochemistry, for chemistry, biophysics, and other STEM majors, it enhances coverage of the basics of biochemistry with relevant medical applications, interactive figures, scaffolded assessment, a Study Guide, adaptive quizzing, activities, and more.
Select a demo to view:
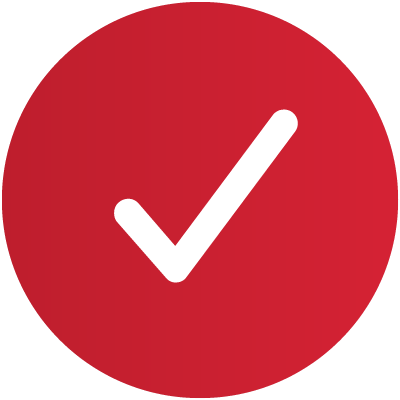